Some Rocks
Jennifer Galloway

Figure 1: A) Geologic map showing location of Sverdrup Basin and Ellef Ringnes Island (after Dewing et al., 2007); B) Jurassic-Cretaceous stratigraphy of the Sverdrup Basin (after Embry, 1991; Dewing and Embry, 2007; Obermajer et al., 2007; Embry and Beauchamp, 2008). Reproduced from Galloway et al. (2015).
This Figure 1 shows the bedrock geology of Sverdrup Basin and Ellef Ringnes Island, a sedimentary basin in Canada’s Arctic (A). The Sverdrup Basin is a 1300-km by 350-km paleo-depocentre in the Canadian Arctic Archipelago. and It contains an up-to-13 km-thick succession of nearly continuous strata spanning from the Carboniferous to Paleogene (B). The sedimentary strata contain a variety of fossils that are of interest to geologists who want to understand what the climate was like during the accumulation of those sediments. This paper of Galloway et al. (2015) uses pollen and spores preserved in the sediments of the Isachsen Formation (B) that was deposited during the Early Cretaceous (145 to 100.5 million years ago). At this time, even though the basin was near its current position in the Arctic, lush forests used to covered the landscape, similar to what now grows in the Pacific Northwest of North America.
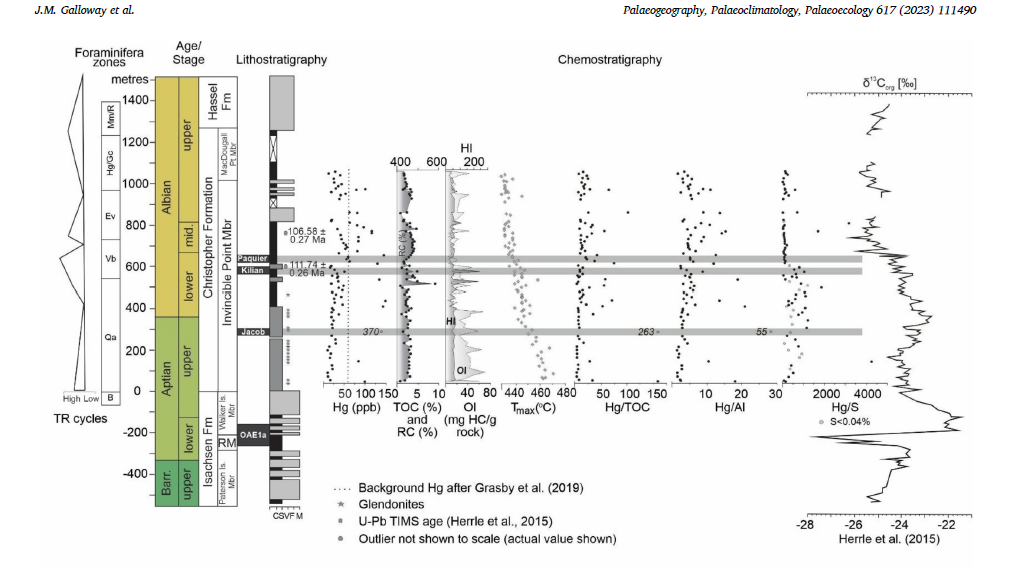
Figure 2. Stratigraphic record of total organic carbon (TOC; wt%) and residual carbon (RC) that comprises a majority of the TOC, Hydrogen and Oxygen indices (HI, OI; mg hydrocarbon/g rock), Tmax (◦C), total Mercury (Hg; ppb), and Hg normalized to TOC, Aluminum (Al), and Sulphur (S) in the Glacier Fiord section on Axel Heiberg Island. Shading denotes the Jacob, Kilian and Paquier sub-levels of OAE 1b. The value is written on an outlier point at 284 m where Hg reaches 370.9 ppb. U–Pb TIMS and the δ13Corg (‰ VPDB) profile after Herrle et al. (2015). Foraminiferal biostratigraphy and sequence stratigraphy after Schröder-Adams et al. (2014). TR – transgressive-regressive; Mm/R – Miliammina manitobensis/Reophax zone; Hg/Gc – Haplophragmoides gigas/Gaudryina canadensis zone; Ev – Evolutinella multiplum zone; Vb – Verneuilinoides borealis zone; Qa – Quadrimorphina albertensis zone; B – barren; RM – Rondon Member; Fm – Formation; Mbr – Member; Is – Island; Pt – Point; C – clay; S –silt; V – very fine sand; F – fine sand; M – medium sand; C – coarse sand. Reproduced from Galloway et al. (2023).
This figure depiction shows how carbon isotopes, Hg, Al, S, and organic matter changes over time in the marine shales of the Aptian to Albian (121.4 to 100.5 million years)-aged Christopher Formation., between 100-121 million years old. The Christopher Formation is a succession of mudstone and siltstone that was deposited during the Early Cretaceous (100.5 to 145 to 100.5 million years ago) in shallow marine seas of the the Sverdrup Basin in the Canadian Arctic (see Figure 1 A, B). The samples were obtained during a field expedition to Glacier Fiord, Axel Heiberg Island in 2011.
During deposition of the Christopher Formation, several globally important climatic and marine changes occurred. These changes were due to emission of the carbon isotope 12C into the atmosphere by large scale volcanism. This carbon injection perturbed the global carbon cycle and is manifesting as a negative isotopic excursion. The injection of substantial quantities of carbon into the atmosphere caused global warming. This global warming led to increased primary production in global oceans, and the organisms preferentially took up the isotope 12C because it is lighter than 13C. Upon decay of the organisms, oxygen was consumed, and global oceanic anoxic events (OAE) were caused. In the low oxygen settings, much of the decayed organic matter was preserved, along with its isotopic carbon signature, and the Christopher Formation records the negative carbon isotopic excursions that are indicative of two of these global events – OAE 1a and OAE1b, the latter of which is sub-divided into multiple sub-events, three of which are preserved in the record from the Christopher Formation (the Jacob, Kilian, and Paquier). These sub-events have French names because they were first described from the Vocontian Basin in France.
At this time, there was massive scale volcanism happening at lower latitudes, as well as in the Arctic. The Arctic volcanism was called the High Arctic Large Igneous Province (the HALIP). Mercury (Hg) has been used as a tracer for volcanism because it may be directly released from volcanoes, or, it is released with magma interacts with host rocks that are high in Hg. Therefore, we assessed Hg in the shales of the Christopher Formation to find out if Hg emitted from HALIP volcanism might have contributed to the carbon isotopic excursion of global oceanic anoxic events (OAE)OAE[GJ1] 1a and OAE1b. Even though these are global events, and Hg signals are often global, we thought that looking close to the possible source made sense.
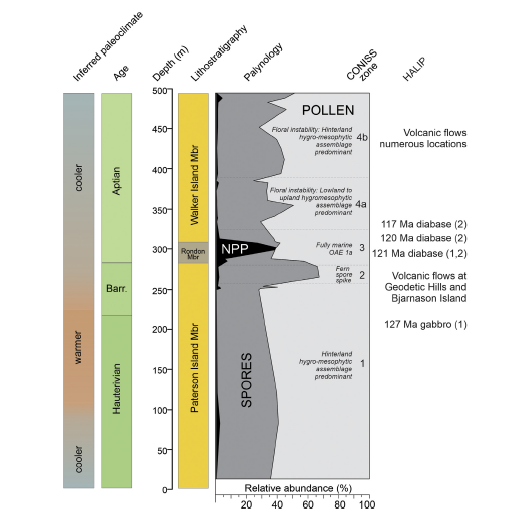
Figure 3. Summary diagram showing the relative abundance of spores, pollen, and non-pollen palynomorphs (NPP), stratigraphic palynological zones delineated using CONISS (Stratigraphically constrained cluster analysis using incremental sum of squares) (see Figure 7 Galloway et al., 2022) in the Isachsen Formation (see Figure 1) at Glacier Fiord, Axel Heiberg Island. palynological events, HALIP (High Arctic Large Igneous Province) events, and inferred paleoclimate during deposition of the Isachsen Formation are also shown. References for HALIP ages are (1) Evenchick et al. (2015) (2) Dockman et al. (2018). References for volcanic flows in Galloway et al. (2022). Reproduced from Galloway et al. (2022).
The Cretaceous Period (145 to 66 to 145 million years ago) is generally considered to have been a time of warm and equable greenhouse climate. This is attributed mainly to high partial pressure of carbon dioxide (~700->4000 ppmv) as a result of elevated background rates of volcanic degassing. However, recent research shows that a number of cooling events punctuated otherwise warm climatic conditions of this interval in the planet’s history. These cooling events may even have caused transient glacial conditions in high northern latitudes. The forcing mechanisms of some Cretaceous climatic perturbations may have been related to carbon drawdown associated with the construction and destruction of one or more large igneous provinces (LIPs). The release of CO2 associated with emplacement of LIPs coupled with weathering of newly extruded basalt may have led to elevated nutrient levels and planktonic productivity in global oceans multiple times during the Cretaceous, periodically resulting in widespread ocean anoxia (for example, see Figure 2). The ensuing carbon burial associated with ocean anoxic events (OAEs) and silicate weathering of newly exposed LIPs are important mechanisms of drawdown of atmospheric carbon dioxide that, in the absence of replenishment, ultimately led to transient global cooling. Thus, LIPs are important drivers for sequences of biogeochemical events that affect global climate. The High Arctic Large Igneous Province (the HALIP) was a protracted event lasting more than 40 million years and is probably the least studied of all known LIPs.
Polar regions are highly sensitive to changes in climate forcing and are important in global climate feedback mechanisms, at present and in the geological past. High northern latitudes were covered with dense coniferous forests during the Early Cretaceous despite extreme photic seasonality. The composition and extent of terrestrial vegetation plays an important role in climate feedback, but information on the role and response of high northern latitude terrestrial plant communities to Mesozoic climate variability is sparse relative to lower latitudes. Moreover, few studies have evaluated the effects of LIPs on terrestrial vegetation despite the importance of terrestrial ecosystems in the carbon cycle, and none have explored the effects of the HALIP on Arctic forests.
This diagram shows how the relative proportion of spores, pollen, and NPP changed over time in the Isachsen Formation. The Isachsen Formation is a succession of marine and terrestrial sandstones, marine mudrock, and coal that was deposited in the Sverdrup Basin in the Canadian Arctic during the a part of the Early Cretaceous (145 to 100.5 million years ago). At this time, lush forests covered the Arctic. These communities were similar to what grows today in the Pacific Northwest of North America. The figure shows how these lush forests were disturbed during the Barremian (Barr.; 125.77 to 121.4 million years ago) by the first volcanic flows of the HALIP that occurred approximately 127.8 to 120 million years ago. This ecological disturbance is manifested by a spike in fern spores (where fern spores increase to make up ~70% of all pollen and spores).
Environmental change can cause conversion of temperate forests into heaths due to disturbance-mediated increases in paludification, nutrient sequestration, release of allelochemicals and contaminants, and soil acidification that cause conifer regeneration failure. The dominance of ferns following disturbance is the result of their tolerance of ecological stress, including the ability to grow on strongly leached and/or nutrient poor or metal-enriched soils, their tolerance of low-light conditions, and certain life-cycle traits such as gametophytic selfing and wind dispersal of spores; together these traits permit their rapid invasion of and growth in disturbed habitats. Fern spore spikes are commonly documented in the geological record associated with large-scale disturbance, including those related to LIP magmatism. Crises at the end-Permian, end-Triassic, and end-Cretaceous demonstrate similar successions of recovery phases of terrestrial vegetation, characterized by a bloom of opportunistic taxa followed by a pulse of pioneer communities and finally recovery of plant communities. Ferns are the most common pioneer taxa, although bryophyte spores may also provide this signal. Geologically brief fern spikes are interpreted to represent the pioneering recovery stage by ferns and fern-allies following collapse of arboreal communities associated with widespread disturbance. This work shows that ecological disturbance of terrestrial plant communities by the HALIP was severe.
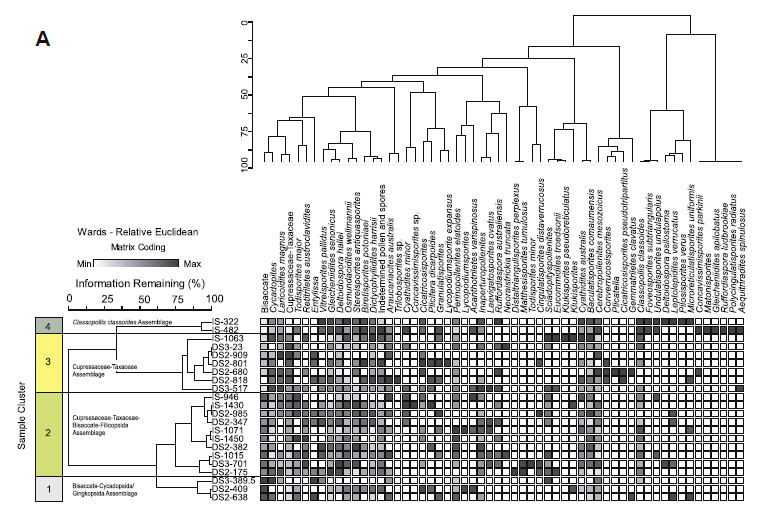
Figure 4. Combined Q- and R-mode cluster analysis of the relative abundance of all spores and pollen identified in the Hoodoo Dome H-37 oil and gas well drilled on southern Ellef Ringnes Island. Reproduced from Galloway et al. (2013).
Figure 4 is the output of two types of cluster analysis – Q mode (samples) and R mode (variables) of the relative abundance of all spores and pollen identified in the Hoodoo Dome H-37 oil and gas well drilled on southern Ellef Ringnes Island. In this case samples represent strata from different geological formations (Sandy Point, Jameson bay, Deer Bay, Ringnes, Christpher formations) and members (Paterson Island and Walker Island members of the Isachsen Formaition) of Jurassic and Cretaceous age from the Sverdrup Basin. The variables represent pollen and spore taxa. The purpose of this multivariate statistical approach was to determine different assemblages of pollen and spores to determine groups of plants that grew together in the environment of the Canadian Arctic at this time. (R mode). Four palynoassemblages (HD-1 to HD-4) are identified. The purpose was also to determine if those assemblages were more or less common in certain geological formations (Q mode). We found that certain assemblages (e.g., HD-4) are more common in older strata. How the relative proportion of the assemblages change over time are shown in Figure 5.
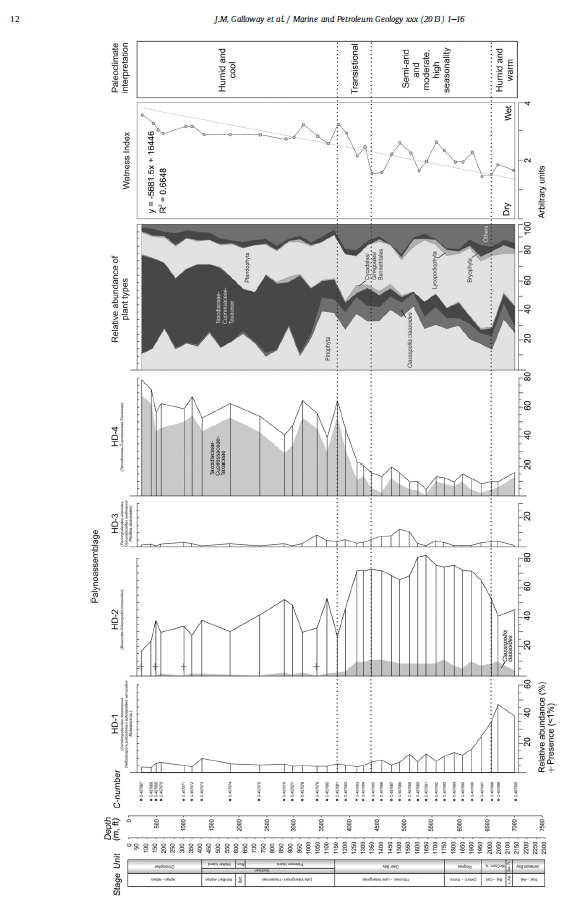
Figure 5. Relative abundances of Palynoassemblages HD-1 through HD-4 preserved in the Hoodoo Dome H-37 oil and gas well, southern Ellef Ringnes Island, plotted stratigraphically. Palynoassemblages derived from combined Q- and R-mode cluster analysis of relative abundance of all pollen. Reproduced from Galloway et al. (2013).
Figure 5 takes the assemblages of pollen and spores as determined from R-mode cluster analysis (Figure 4) and plots those assemblages over time to determine how terrestrial plant communities changed during the Late Jurassic to Early Cretaceous interval (161.5 to 100.5 million years ago) in the Canadian Arctic. The ecological preferences of nearest living relative of these ancient plants are used to infer how the climate change affected changes in terrestrial plant communities. The figure shows that there is a marked change during the Early Cretaceous from a seasonally arid climate to more humid and cool climatic conditions. This climate shift resulted in the demise of some taxa, most notably, Classopollis classoides, a pollen taxon produced from the Cheirolepidiaceae, an extinct group of coniferous plants belonging to the Class Pinopsida (the “pines”). The parent plants that produced Classopollis classoides were xerophytic or drought resistant and thermophilous, requiring at least a subtropical climate. This climatic shift to cooler and more humid conditions also promoted the expansion of Cupressaceae (cedar family) trees in the Canadian Arctic at this time.
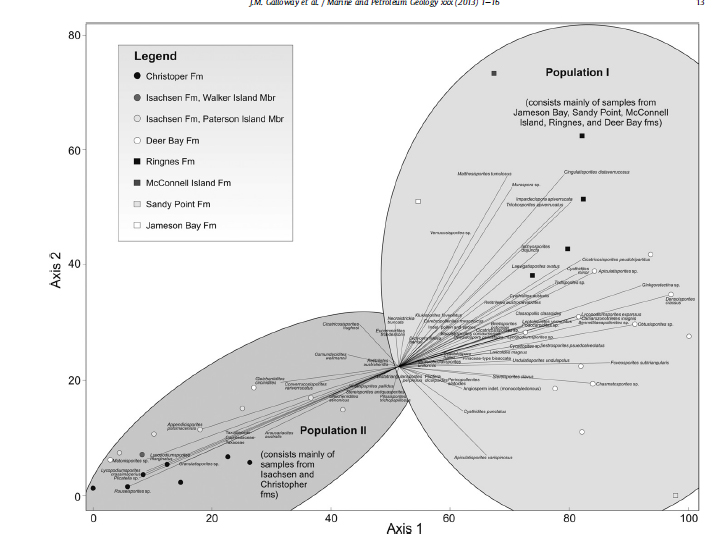
Figure 6. Non-metric multidimensional scaling ordination biplot of relative abundance of pollen and spores identified in Hoodoo Dome H-37. A proportion of maximum scaling was used. Reproduced from Galloway et al. (2013).
Figure 6 shows the result of a statistical method to reduce dimensionality in a multi-variate dataset. The figure shows that two main populations of samples and plant taxa occur in strata of Late Jurassic to Early Cretaceous age in the Canadian Arctic. This major change occurs in the Early Cretaceous and is illustrated stratigraphically in Figure 5.
Dewing, K., Embry, A.F., 2007. Geological and geochemical data from the Canadia
Arctic Islands. Part I: stratigraphic tops from Arctic Islands' oil and gas exploration boreholes.
Geological Survey of Canada. Open File 5442, 7 p., 1 CD.
Dewing, K., Turner, E., Harrison, J.C., 2007. Geological history, mineral occurrences
and mineral potential of the sedimentary rocks of the Canadian Arctic Archipelago.
In: Goodfellow, W.D. (Ed.), Mineral Deposits of Canada: A Synthesis of
Major Deposit-Types, District Metallogeny, the Evolution of Geologic Provinces, and Exploration
Methods. Geological Association of Canada, Mineral Deposits
Division. Special Publication 5, pp. 733e753.
Dockman, D. M., Pearson, D. G., Heaman, L. M., Gibson, S. A., & Sarkar, C. (2018). Timing and
origin of magmatism in the Sverdrup Basin, Northern Canada—Implications for lithospheric evolution
in the High Arctic Large Igneous Province (HALIP). Tectonophysics, 742–743, 50– 65.
Embry, A.F., 1991. Mesozoic history of the Arctic Islands, Chapter 14. In: Trettin, H.P. (Ed.),
Inuitian Orogen and Arctic Platform: Canada and Greenland, pp. 369e433. Geological Survey of
Canada, Geology of Canada 3.
Embry, A.F., Beauchamp, B., 2008. Chapter 13 Sverdrup Basin. In: Miall, A.D. (Ed.), Sedimentary
Basins of the World, 5, pp. 451e471.
Evenchick, C. A., Davis, W. J., Bédard, J. H., Hayward, N., & Friedman, R. M. (2015). Evidence for
protracted High Arctic large igneous province magmatism in the central Sverdrup Basin from
stratigraphy, geochronology, and paleodepths of saucer-shaped sills. Bulletin of the Geological
Society of America, 127(9–10), 1366–1390.
Galloway, J. M., Fensome, R. A., Swindles, G. T., Hadlari, T., Fath, J., Schröder-Adams, C., Herrle,
J. O., & Pugh, A. (2022). Exploring the role of High Arctic Large Igneous Province volcanism on
Early Cretaceous Arctic forests. Cretaceous Research, 129.
Galloway, J. M., Grasby, S. E., Wang, F., Hadlari, T., Dewing, K., Bodin, S., & Sanei, H. (2023). A
mercury and trace element geochemical record across Oceanic Anoxic Event 1b in Arctic Canada.
Palaeogeography, Palaeoclimatology, Palaeoecology, 617(October 2022).
Galloway, J. M., Sweet, A. R., Swindles, G. T., Dewing, K., Hadlari, T., Embry, A. F., & Sanei, H.
(2013). Middle Jurassic to Lower Cretaceous paleoclimate of Sverdrup Basin, Canadian Arctic
Archipelago inferred from the palynostratigraphy. Marine and Petroleum Geology, 44, 240–255.
Galloway, J. M., Tullius, D. N., Evenchick, C. A., Swindles, G. T., Hadlari, T., & Embry, A.
(2015). Early Cretaceous vegetation and climate change at high latitude: Palynological evidence
from Isachsen Formation, Arctic Canada. Cretaceous Research, 56, 399–420.
Herrle, J. O., Schröder-Adams, C. J., Davis, W., Pugh, A. T., Galloway, J. M., & Fath, J. (2015).
Mid- arctic stratigraphy, climate, and oceanic anoxic events. Geology, 43(5), 403–406.
Obermajer, M., Stewart, K.R., Dewing, K., 2007. Geological and geochemical data from the Canadian
Arctic Islands. Part II: Rock-Eval data. Geological Survey of Canada. Open File 54590, 1CD.
Schröder-Adams, C. J., Herrle, J. O., Embry, A. F., Haggart, J. W., Galloway, J. M., Pugh, A. T., &
Harwood, D. M. (2014). Aptian to Santonian foraminiferal biostratigraphy and paleoenvironmental
change in the Sverdrup Basin as revealed at Glacier Fiord, Axel Heiberg Island, Canadian Artic
Archipelago. Palaeogeography, Palaeoclimatology, Palaeoecology, 413, 81–100.